A New Technology Enables Manipulation of Neurons in Peripheral Organs and Reveals a Mechanism of Appetite Suppression
A New Technology Enables Manipulation of Neurons in Peripheral Organs and Reveals a Mechanism of Appetite Suppression
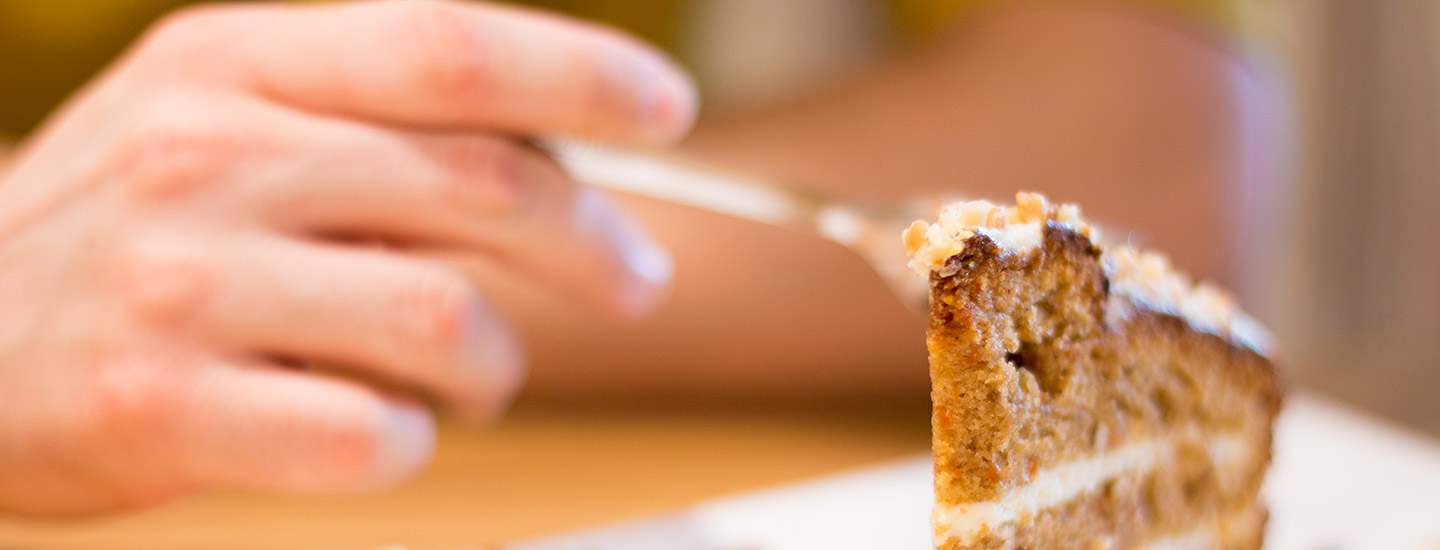
A research team led by a 2018 BBRF Young Investigator, Sung Il Park, Ph.D., reports that it has developed and tested a new technology enabling unprecedented exploration of nerve-cell function inside organs of the body outside of the brain.
The new technology, called optoelectronics, uses tiny wireless implantable devices to manipulate the activity of individual nerve cells in the organs of awake, freely moving animals. This makes possible experiments with the power to reveal the specific (and often multiple) functions of different kinds of nerve cells in the body's periphery. Such knowledge, which is still spotty, is a vital predicate of future experiments to test new therapeutic concepts.
As reported in Nature Communications, the novel wireless devices created by Dr. Park and colleagues at Texas A&M University were successfully implanted in the stomachs of laboratory mice, and enabled the team to discover an unexpected mechanism for suppressing appetite. This is a hint of the technology's potential utility in identifying new treatment targets—in this application, for obesity and eating disorders.
In the early 2000s, Karl Deisseroth, M.D., Ph.D., with help from two BBRF Young Investigator grants, pioneered a revolutionary technology called optogenetics, whose reach the newly reported technology significantly extends.
With optogenetics, Dr. Deisseroth, now a member of BBRF's Scientific Council and winner of the 2013 BBRF Goldman-Rakic Prize, discovered a way to switch individual neurons in the brain "on" and "off," by genetically adapting various neuronal types to activation by specific wavelengths of light. The technology, soon adopted by neuroscientists worldwide, is now a staple of brain research, and has revealed much about how the brain functions—and malfunctions—in illnesses such as depression, anxiety, and PTSD.
Like optogenetics, the wireless "optoelectronic" technology invented by Dr. Park and colleagues can provide deep insights into what goes awry in illness by obtaining new knowledge about how cells and circuits function. In contrast with existing optogenetics approaches, which are used to explore neural and circuit function in the brain, Dr. Park's wireless technology is designed to work in the body's peripheral organs and to probe the complex highways of nerves that connect those organs with the brain.
Optogenetics is limited to the brain due to constraints associated with delivering light via fiberoptic technology to the body's periphery. "Body tissues typically lack a stable interface for securing fiberoptics," Dr. Park's team notes. Moreover, they explain, optogenetic probes, being relatively inflexible, will cause "shearing of tissues and nerves during an animal's natural movements."
The new technology has no such constraints. At its heart are extremely small "microscale light-emitting diodes" with soft, highly flexible tethers, which are implanted into the peripheral organ of interest. These devices enable the technology to operate where optogenetics cannot, Dr. Park says. The devices are externally controlled via wireless platforms on a single transmitter that can direct experiments in as many as eight animals (living in adjacent cages) at a time. Testing showed that the devices were effective for a month in freely behaving animals.
In their paper the team explained that one "key priority for research is to attain cell-type and organ-specific manipulations of the vagus nerve, in animals that are awake." The vagus nerve is the body's most important nerve pathway connecting the heart, lungs, and digestive tract with the brain. Its function accounts for the body's remarkable ability to unconsciously regulate breathing, the pumping of the heart, and the breaking down of food into nutrients and waste products.
The focus of Dr. Park's team in their test of wireless optoelectronics were the endings of the vagus nerve within the stomach—known to be important in the regulation of appetite. The specific targeting made possible by optoelectronics enabled the team to concentrate on a type of neuron found in the stomach that is characterized by its expression of a gene called Calca+. They used their wireless device to selectively activate Calca+ neurons which connected with nerve-endings from the vagus nerve in a part of the stomach called the corpus.
Compared with mice in which optoelectronics was not used to stimulate stomach neurons of this type, mice that did receive stimulation via wireless commands showed "robust suppression of food intake during feeding," the team reported. In other words, by activating specific vagus nerve endings in a specific area of the stomach, appetite was suppressed—to the point of almost complete suppression when the stimulation was increased in intensity.
It is widely believed that when the stomach is full and has stretched, information about its stretch is conveyed to the brain by receptors on the vagus nerve. “Our findings suggest that stimulating non-stretch receptors, those that respond to chemicals in the food, could also give the feeling of satiety [fullness] even when the stomach is not stretched,” Dr. Park explains.
The team also discovered that activation of Calca+ vagal nerve endings seems to trigger an aversive reaction which alters an animal's taste preferences. Thus, these early optoelectronics experiments resulted in identification of a role of vagus nerve endings in the stomach in appetite suppression, and a mechanism involving taste-aversiveness which causes the loss of appetite.
The team described the implications: "Identification of pathways that can either suppress or stimulate appetite will have direct clinical importance for potentially developing novel therapeutics for treating appetite disorders."